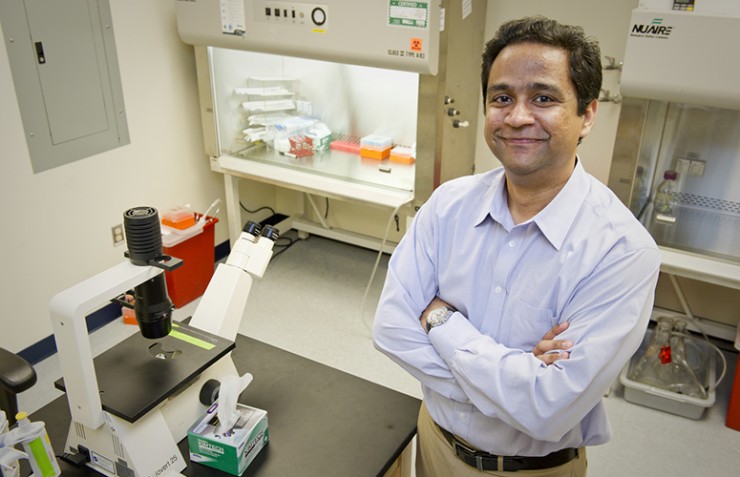
By Thea Singer
News at Northeastern
BOSTON–Metastasis. The very word evokes fear. Defined as the spread of cancer cells from one part of the body to another, metastasis is the cause of approximately 90 percent of deaths among cancer patients. How does metastasis come about? And can we stop it?
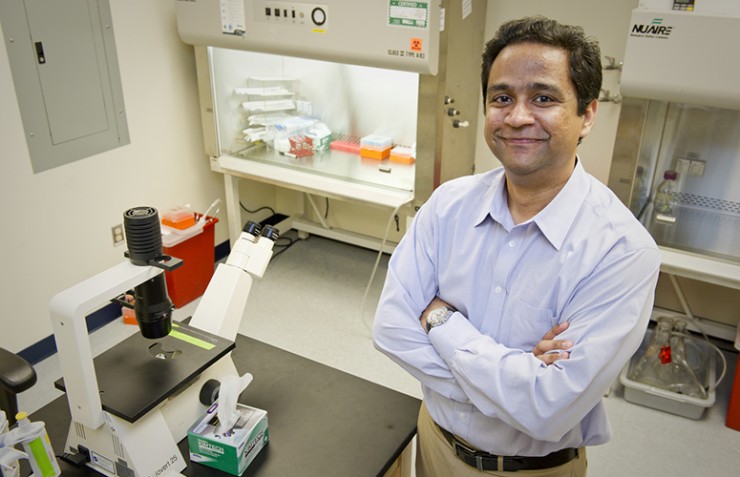
New research from a team led by Northeastern’s Anand Asthagiri, associate professor of bioengineering and chemical engineering, helps to answer those questions. It provides an astonishing look at the biophysical properties that permit breast cancer cells to “slide” by obstacles and travel out of their primary tumor toward a blood vessel that will carry them to a new site.
The paper, published Tuesday in Biophysical Journal, reveals how the abnormal protein-fiber scaffolding of tumors and the agility of the cancer cells themselves come together in a perfect storm to enable the escape. The quantitative method the researchers developed to understand the cells’ sliding ability could also lead to a new way to screen for effective cancer drugs and help diagnose the stage of a cancer early on.
“We were showing that there are different levels of sliding ability, and we measured each one.”
— Anand Asthagiri, associate professor
“We are looking at the interaction between cancer cells’ migrating and this sliding phenomenon, and how that’s influenced by the protein-fiber environments of tumors,” says Asthagiri. “In this paper we show that cancer cells migrating on these protein fibers have a unique ability that enhances their invasion capacity: When they bump into other cells—which the microenvironment is packed with—they slide around them. Normal cells halt and reverse direction.”
An interdisciplinary approach
The researchers’ engineering backgrounds shaped their interdisciplinary approach: They set out to explore the mechanics of the sliding ability as well as its molecular components.
To do so they developed a model environment that mimics protein fibers. First they stamped stripes of a protein called fibronectin on glass plates, making sure to represent various widths. “If you treat a fiber as a cylinder, imagine cutting it and opening it up and laying it flat,” says Asthagiri. “That’s essentially what these long stripes of protein mimicked.” Then they deposited the cells—alternately hundreds of breast cancer cells and hundreds of normal cells—on these fiberlike stripes and used a microscope with time-lapse capabilities to observe and quantify their behavior.
On fibers that were 6 or 9 microns wide—the typical size of fibers in tumors—half the breast cancer cells elongated and slid around the cells they collided with. Conversely, 99 percent of the normal breast cells did an about face.
But why? To understand what gave the cancer cells this remarkable agility, Asthagiri and his colleagues, who included Daniel F. Milano, a former graduate research assistant at Northeastern, introduced “genetic perturbations” into the mix—that is, they inserted certain proteins into the cancer cells and took the same proteins out of the normal cells. Among them was E-cadherin, a sticky protein that enables cells to bind to one another.
“Cancer cells often lack E-cadherin,” says Asthagiri. “When we introduced it genetically, the cancer cells’ ability to slide diminished. And when we took E-cadherin out of normal cells, they acquired some sliding ability once the fibers were wide enough.” Together, the varying widths of the fiber paths and the perturbations produced a wealth of quantitative data about how the cells, both cancerous and normal, behaved under different conditions.
“We weren’t just showing that cells either slide or don’t slide,” says Asthagiri. “We were showing that there are different levels of sliding ability, and we measured each one.”
Multiple applications
Asthagiri’s system is relatively easy to construct and suited for rapid imaging—two qualities that make it an excellent candidate for screening new cancer drugs. Pharmaceutical companies could input the drugs along with the cancer cells and measure how effectively they inhibit sliding.
In the future, the system could also alert cancer patients and clinicians before metastasis starts. Studies with patients have shown that the structure of a tumor’s protein-fiber scaffolding can indicate how far the disease has progressed. The researchers found that certain aggressive genetic mutations enabled cells to slide on very narrow fibers, whereas cells with milder mutations would slide only when the fibers got much wider. Clinicians could biopsy the tumor and measure the width of the fibers to see if that danger point were approaching. “We can start to say, ‘If these fibers are approaching X microns wide, it’s urgent that we hit certain pathways with drugs,” says Asthagiri.
Questions, of course, remain. Do other types of cancer cells also have the ability to slide? What additional genes play a role?
Next steps, says Asthagiri, include expanding their fiberlike stripes into three-dimensional models that more closely represent the fibers in actual tumors, and testing cancer and normal cells together. “There are so many types of cells in a tumor environment—immune cells, blood cells, and so on,” he says. “We want to better emulate what’s happening in the body rather than in isolated cells interacting on a platform.”
(Published with permission from News at Northeastern.)